WSPOS Visual Electrodiagnostics & Eye Movement Recording (EMR) Consensus Statement – 2018
Part 1. Visual Electrodiagnostics
Manca Tekavčič Pompe & Alki Liasis
1. Basic concepts of visual electrophysiology and its role in pediatric ophthalmology
Visual electrophysiology consists of a range of investigations that record bioelectrical activity elicited by visual stimulation. Electrophysiological tests provide objective measures of visual pathway function and due to their noninvasiveness they represent a great diagnostic and prognostic tool for pediatric ophthalmologists. In everyday clinical practice visual electrodiagnostic tests include: EOG (electrooculogram) for retinal pigment epithelium (RPE) evaluation, ERG (electroretinogram) for retinal function evaluation and VEP (visual evoked potentials) for post retinal visual pathway evaluation. Each of the visual electrodiagnostic testing modalities consists of different stimuli to asses a particular subgroup of retinal cells function or a specific part of visual pathway. Stimuli vary in stimulation mode (flash vs. pattern), appearance of the stimulus (reversal vs. onset), color (black-white vs. colored), size (different check sizes), intensity and duration of flash. Electrophysiological testing is very suitable for pediatric population, since testing is non-invasive, does not require any behavioral feedback, though the child’s attention to stimuli is very important for some tests while others require little cooperation. There are some child-friendly modalities of commercially available visual electrodiagnostic systems, such as hand-held stroboscopes and ERGs, skin electrodes (instead of corneal electrodes) and promising new systems for full-field ERG recording with a hand-held mydriasis free device. In order to achieve a global consistency of the recordings and interpretation of the findings, international standards and recommendations were published by International Society for Clinical Electrophysiology of Vision (ISCEV).
2. The electrooculogram (EOG)
EOG measures the existing resting electrical potential between the cornea and Bruch’s membrane. The typical standing potential value is 6 millivolts (mV) with a positivity at the cornea. The EOG is displayed as a voltage time plot that enables the graphical display of eye movements. When retinal pigment epitheliopathy is suspected in a maculopathy, EOG has a potentially diagnostic role. Light and dark adaption results in modulation of the EOG amplitude. The light-insensitive component accounts for the dark trough and is dependent on the integrity of the retinal pigment epithelium (RPE). The light-sensitive component is the slow light rise of the EOG and is generated by the depolarization of the basal membrane of the RPE. Since the dark trough and light rise occurs around 8 minutes after a change in light adaption a subject is required to make reproducible saccades for 10-15 minutes in the dark followed by 10-15 minutes in the light. As a result, this investigation is less applicable in young and uncooperative children. The EOG is often used in the diagnostics of maculopathies (for example Best disease). The amplitude of the dark trough and light rise EOG is presented as a ratio (the Arden ratio). Arden ratios of 1.8 or more are considered normal.
3. The electroretinogram (ERG)
ERG’s evoked by a variety of stimuli can evaluate separately cone and rod photoreceptor function as well as distinguish photoreceptor from inner retinal function.
Scotopic (rod) responses are isolated by dark-adaptation for a minimum of 20 minutes according to ISCEV standards followed by a visibly white stimulus with an intensity of 0.010 photopic cd·s·m-2 as a single flash or 10 Hz flicker.
The response is primarily rod driven response from on bipolar cells. In a dark-adapted state a bright flash stimulus of 10 cd·s·m-2 evokes a combined response with enhanced ‘a’ waves.
Photopic (cone) responses can be obtained either before or after dark-adaptation. Cone photoreceptor function is primarily measured under light-adapted conditions for at least 10 minutes using a standard background luminance of 30 photopic cd·s·m-2. Stimuli used include either single flash or 30 Hz flicker stimuli as rods cannot follow a flicker stimulus greater than 20 Hz.
The flash ERG (fERG) represents the net summation of retinal activity as the whole retina is stimulated.
A pattern ERG (PERG) evoked by equal numbers of reversing black and white elements (usually checks) can differentiate between distal macular and ganglion cell function.
The multifocal (mfERG) allows local ERG responses to be recorded simultaneously from many regions of the retina.
Black and white hexagons are flashed on and off in an M-sequence, the waveforms are mathematical constructs, not a group of focal ERGs.
fERG responses consist of:
• a-wave: the first major negative component related to the hyperpolarization of the photoreceptors (outer retina) in response to light.
• b-wave: positive component, derived mostly from ON-bipolar and Mueller cells (inner retina).
• Oscillatory potentials: a series of waves between a- and b- waves, derived probably from bipolar/amacrine cells and inner plexiform layer (inner retina).
• c-wave: a slow, positive component derived from RPE and photoreceptors. PERG responses to pattern reversal checks consist of:
• P50 wave: the positive wave that mainly represents localized macula photoreceptor function.
• N95 wave: the negative wave that mainly reflects ganglion cell function.
• mfERG is a topographic measure of retinal electrophysiological activity.
• Used to localize spatial damage of the retina
4. The visual evoked potentials (VEP)
VEP is a response to visual stimuli that is recorded at the occipital cortex and mainly reflects post retinal visual pathway function. However, it can be influenced by general retinal dysfunction and maculopathy, therefore it is clinically very important to combine VEP with ERG/PERG to obtain additional information on macular function.
VEPs are recorded binocularly and monocularly from 3-5 electrodes positioned occipitally, over the visual cortex. The stimulation depending on the stimulus consists of full-field stimulus and, where possible, half-field stimulation, to discriminate optic nerve, chiasmal, and hemisphere anomalies.
Different types of stimulation can be used: pattern reversal VEP, pattern onset VEP and flash VEP.
Pattern reversal VEP stimulus consists of black and white checks of different sizes (typically from 400` to 6.25`) generally reversing at a speed of 1.8Hz. ISCEV standards using test checks subtending 60 and 15 minutes of arc° ± 20%. The response consists of three waves N75, P100 and N135 (N stands for negative wave, P for positive), the P100 being the hallmark of the response and relatively stable throughout the lifespan but has a rapid decreased in latency over the first 3 months of life.
Pattern onset VEP is a stimulus less sensitive to eye movements and lack of attention. The response consists of CI positivity around 90 ms, CII negativity around 110 ms and CIII positivity around 180-200 ms. Because of its complexity and different maturation throughout the lifespan, the onset VEP response is not optimal for monitoring of the changes throughout life. Responses can as with reversal be evoked by a range of different size stimuli.
Flash VEP is very robust and efficient especially in babies to get a basic idea about visual pathway function, however the combined information using flash ERG, flash VEP and pattern reversal VEP gives a clinician additional insight into visual pathway function.
5. Most common pediatric ophthalmologist’s questions visual electrodiagnostics can help to answer
• Can the baby/ this very developmentally delayed child see and in what size range is the visual acuity?
Flash VEP gives the clinician an idea of the integrity of the general visual pathways, responses to different check sizes of pattern reversal VEP tell the clinician about macular pathway function and quantity of potential visual acuity – good visual acuity means good sized response to smallest checks (6.25´), whereas poor visual acuity means small response to the largest checks (400´). The range of check sizes employed depends on instrumentation, lab setup and normative data
• Is the nystagmus in a baby/child due to retinal disease or chiasmal crossing pathology?
Full field ERG can answer the question about retinal dystrophies (cone/rod, cone driven, rod driven response), VEP can demonstrate crossing asymmetry such as excessive crossing of the retinal fibers in chiasma (as in albinism) and insufficient crossing of the retinal fibers in chiasma (as in achiasmia), which can all be causes of nystagmus.
• What type of retinal dystrophy does the child have? Is it progressive?
Full field ERG with additional tests can answer the question which part of the retina is primarily affected. If the inner retina is dysfunctional but the photoreceptors are functional the ERG will show a reduced ‘b’ wave. The follow-up recordings can help the clinician demonstrate weather the condition is stationary or progressive.
• Do certain drugs affect visual function in this child?
The effect of retinotoxic drugs can be demonstrated by pattern reversal ERG and full-field ERG, where the initial values are of great importance. Follow-up recordings demonstrate weather the drug has affected retinal function or not. The effect of neurotoxic drugs can be demonstrated using VEP. Comparison of multiple recordings can also give additional information about the damage certain drug has made.
• Monitoring of visual pathway function in a child with optic nerve disease.
A combined use of pattern reversal ERG and VEP is of great importance in these cases, since the N95 PERG wave is primarily retinal ganglion cells driven response and VEP demonstrate post retinal visual pathway function.
• Chiasmal abnormalities in a child (such as chiasmal tumor).
VEP is the most important visual electrodiagnostic method in these cases. It is very important to use at least 3 electrodes over the occipital cortex to be able to detect any asymmetry. The use of half-field stimulation in addition to full-field stimulation gives additional information about visual pathway function. It is also suggested to use a variety of stimulus modalities (flash and pattern) as one may not detect chiasmal dysfunction but another may.
• Is visual pathway function compromised due to craniosynostosis in the child?
A baby with craniosynostosis can be evaluated electrophysiologically to determine any possible visual pathway function deficiencies by using flash VEP, pattern reversal VEP and onset VEP. The visual electrodiagnostics is also useful to monitor the status after surgical treatment.
• Functional visual loss in an older child? Malingering?
Visual electrodiagnostics have an important role in demonstrating functional visual loss and malingering in otherwise healthy child with no identifiable ocular pathology who claims to have poor vision and performs visual field testing badly (often demonstrating concentric visual loss). Combined PERG and VEP responses should be completely normal and if there is any doubt also full-field ERG responses are added and show no abnormality.
Figures 1 and 2 show ERG and VEP flow-charts to help the clinician decide which visual electrodiagnostic test to use in a certain suspected pathology.
Selected References
1. Paul A. Constable PA, Bach M, Frishman LJ, Jeffrey BG, Robson AG for the International Society for Clinical Electrophysiology of Vision. ISCEV Standard for clinical electro-oculography (2017 update). Doc Ophthalmol 2017;134:1–9.
2. McCulloch DL, Marmor MF, Brigell MG, Hamilton R, Holder GE, Tzekov R, Bach M. ISCEV Standard for full-field clinical electroretinography (2015 update). Doc Ophthalmol 2015;130:1–12.
3. Bach M, Brigell MG, Hawlina M, Holder GE, Johnson MA, McCulloch DL, Meigen T, Viswanathan S. ISCEV standard for clinical pattern electroretinography (PERG) – 2012 update. Doc Ophthalmol 2012;126:1–7.
4. Hood DC, Bach M, Brigell M, Keating D, Kondo M, Lyons JS, Marmor MF, McCulloch DL, Palmowski-Wolfe AM for the International Society For Clinical Electrophysiology of Vision. ISCEV standard for clinical multifocal electroretinography (mfERG) (2011 edition). Doc Ophthalmol 2012;124:1–13.
5. Odom JV, Bach M, Brigell M, Holder GE, McCulloch DLL, Mizota A, Tormene AP. ISCEV standard for clinical visual evoked potentials (2016 update). Doc Ophthalmol 2016; 133(1):1–9.
6. Thompson DA, Liasis A. Visual electrophysiology: how it can help you and your patient. In: Taylor&Hoyt´s
Pediatric ophthalmology and strabismus. Lambert S, Lyons C, eds. Elsevier 5th edition, 2016: 68-75.
7. Pompe MT, Kranjc BS, Brecelj J. Visual evoked potentials to red-green stimulation in schoolchildren. Vis Neurosci 2006; 23 (3-4): 447-51.
8. Tekavcic-Pompe M, Stirn-Kranjc B, Brecelj J. Optic neuritis in children – clinical and electrophysiological follow-up. Doc Ophthalmol 2003; 107 (3): 261-70.
Part 2. Eye Movement Recording (EMR)
Richard Hertle
1. Introduction
Eye movement recording has become an important clinical and research tool in the evaluation, diagnosis and management of neurologic and ophthalmologic disorders. The clinical values of EMR have increased dramatically in the last 20 years and include; physical improvements in recording and analysis techniques, application in infants and children and understanding of several different types of eye movements. It is now easy to quantitate eye movements for clinical and research purposes and well as identification of mild, subclinical disorders previously unknown. Using eye-movement recordings, accurate, repeatable diagnoses can be made of almost disorders of ocular motility including; every strabismus type and differentiation of all forms of nystagmus; e.g., infantile nystagmus syndrome (previous “congenital nystagmus”), fusion maldevelopment nystagmus syndrome (previous “latent nystagmus), nystagmus blockage syndrome, pendular nystagmus of the nucleus of the optic tract associated with either INS or FMNS, spasmus nutans syndrome, vestibular nystagmus and all other types of acquired nystagmus.
2. History
The instrumentation for recording all types of eye movements was derived from that used originally to record vestibular nystagmus. Purkinje noted eye movements by visual observation in 1825 and E. Darwin by palpations of the eyes in 1794. Studies of eye movements by visual observation were described by Java1 in 1879. The earliest mechanical methods of recording eye movements were proposed by Raehlmann in 1878 who used one end of a lever attached to the globe and with the other end of the lever, recorded the transmitted eye motions, on a moving smoked drum. The technique further improved when small cups resembling contact lenses were attached to the cornea. In 1899, Orschansky fixed a small mirror to the cup on the eye and used a beam of light to project the reflected eye movements onto a screen. Photographic analysis of nystagmus was introduced by Dodge and Cline in 1901 and 1903. In 1913 Coppez used early cinematography. Schott and Meyers in 1922 measured electrical potentials with skin electrodes attached near the eye. This technique has been the basis of modern electronystagmography or electrooculography (ENG or EOG).
Non-contact optical methods are currently the most popular. The use of infrared light reflected from the eye which is sensed by specially designed optical sensors remains common. A voltage is generated from the difference in reflection between the sclera and iris as the eye moves and is the basic output to extract eye rotation information. Video-based eye trackers typically use one or multiple Purkinje images and the center of the pupil as features to track eye movement over time. These optical methods, particularly those based on video recording, are now widely used and are favored for eye-movement analysis. They are especially useful in infants and children, being non-invasive and inexpensive. These so-called double Purkinje image (DPI) eye trackers reach high resolution, accuracy, and bandwidth. Videooculography (VOG), defined as the use of these methods for dynamic measure of eye movements, became feasible with the rapid development computer-based automatic image processing. This progress is mainly reflected in the frame rates being processed online and in the robustness and the accuracy of the marker detection algorithms. The magnetic search-coil technique, developed by Robinson and Collewijn et al., was extended by Collewijn et al. and Kasper and Hess to cover 3-D movements.
3. The Electrooculogram, Electronystagmogram (EOG, ENG)
The simplest method for measuring human eye movements is based on the feature that the human eye is an electrical dipole. The retina is more negative than the cornea. The potential difference of about 6 millivolts results from the electrical activity of photoreceptors and neurons in the retina. However, the EOG measures the eye dipole as it rotates. This causes small differences between the electrical potential at the skin surface next to the eye depending on eye position. A rightward eye movement will increase the surface potential at the temporal canthus and decrease it at the nasal canthus of the right eye. The voltages are usually referenced to a third electrode that is generally placed at the forehead or one of the mastoid processes or on the earlobe. To simultaneously record vertical eye movements, two additional electrodes must be placed below and above the eye. Vertical EOG signals are less reliable than horizontal ones signals due to lid artifacts. The resolution of both horizontal and vertical EOG signals is limited by electromagnetic field noise in the environment, thermal noise generated by the input resistance of the amplifier and the contact resistance of the skin electrodes, and capacitive noise due to electrical activity of muscles and neurons. To lower the contact resistance, the skin should be cleaned with alcohol. Electrodes should be made of relatively nonpolarizeable material such as silver-silver chloride or gold and applied with a conductive paste. Subjects are instructed to avoid any movements except eye movements. Changes of the dark adaptation level induce slow drifts of the corneo-retinal potential which are superimposed on the EOG signal. Since both the EOG and ERG measure the corneo-retinal potential, the standards of ERG recordings are also recommended for EOG recordings. The spatial of resolution of EOG is ~1 degree, temporal resolution ~40 Hz, vertical recording is confounded by blink artifact, noise is 1 degree or more, setup is slow, and calibration is needed. Cost is ~ $500.00.
4. Infrared Reflection Device (IR)
IRD’s measure the differences in intensity of infrared light reflected from across the surface of the eye at a fixed location from the eye. Light intensity is measured with photo diodes that have a high temporal resolution. The distance between eye and photoreceptors is in the range of 24 mm. At such small distances, the differences in the intensity between the different photodiodes depend mainly on the position of iris and pupil, which reflect less light than the surrounding sclera. IRD’s are very sensitive to relative translations of the photodiodes and the eye because they do not evaluate the angle, but only the intensity of the reflection. For an eye radius of 1.25 cm, a translational error of 1 mm will lead to an eye position error of almost 5. The system must therefore be either firmly attached to the head or the head firmly fixed in relation to the system. IRD’s have a much lower noise level than EOG, but they suffer from eyelid artifacts that critically depend on the position of the photodiodes. These lid artifacts may increase dramatically if the device is not properly adjusted in front of the eye. Lid artifacts are more pronounced for vertical than for horizontal eye movements. Moreover, the position of the photodiodes is also critical for the system linearity. Due to these features, optimal adjustment of the device requires that the experimenter carefully controls the eye-position signal of the IRD and compares it with the eye movements. The spatial resolution is ~0.1 degree, temporal resolution is 100 – 500 Hz, vertical recording is confounded by blink artifact and the intrinsic difficulty in distinguishing lid movement from eye movement. Setup is fast but calibration is necessary. Linearity is a major problem with non-linearity occurring at 15 to 20 degrees. Cost is moderate, ~ $4,000.
5. Scleral Search Coil
The scleral search-coil system measures the voltages in one or two coils induced by two or three rapidly oscillating magnetic fields. The coils are molded in a soft contact annulus that is attached to the eyeball. Three pairs of large coils, mounted in a cubic frame, generate the magnetic fields. The subject’s head is positioned at its center. The field coils should be large, because the homogeneity of the magnetic field is crucial for the precision of the measurement. With pairs of square-shaped coils, arranged in a cubic configuration, the inhomogeneity inside of a central test cube stays below 5% when the edge length of the test cube approaches one fifth of the edge length of the field coil. The voltage induced by one of the magnetic fields in the scleral search coil is proportional to the projection of the coil vector (defined as the vector orthogonal to the effective coil plane) onto the magnetic field vector. Thus, the three voltages induced by three orthogonal magnetic fields form the vector components of the coil vector expressed in field coordinates. Methods to compute the 3-D eye orientations from these six signals are then employed. Systems with three magnetic fields can be objectively calibrated, i.e. their calibration does not rely on accurate fixation of targets at different positions, as most other recording techniques. Only a single fixation target is needed in order to determine the orientation of the coil with respect to the eye. Another important advantage of 3-field systems over 2-field systems is that the orientation of the coil vector can be determined without knowledge of the actual inductance of the scleral search coil. With the search-coil technique, the inherent system noise of horizontal and vertical eye position has been estimated to be on the order of 0.5 min of arc (0.0083). The system resolution is a very important parameter; it determines the smallest eye movement that can be detected. However, to compare the metrics of eye movements between different subjects or with a stimulus-defined requirement the accuracy is more important than the system noise. The system accuracy of search coils depends mainly on the quality of the calibration. Due to its large signal to noise ratio and reliability, the search-coil technique has been the generally accepted reference standard for eye movement recordings for 30 years. However, the disadvantages, connected with the invasiveness of the method, have also been recognized. The search coil not only measures eye movements, but also affects them. Some authors have found that saccades last longer (by about 8%) and become slower (by about 5%) when subjects wear search coils in both eyes than when they do not. It was also shown that the eye torsion, when evaluated with the search coil, depends on the orientation of exit point of the connecting line from the search coil. Other disadvantages of the scleral search coil are that wearing the coil may lead to drying, and temporal deformations of the cornea, and reduced visual acuity in the eye with the search coil. Therefore, the manufacturer of the search coil limits wearing time to 30 minutes. The coil spatial resolution is ~0.01 degree, temporal resolution at least 1000 Hz. Vertical and torsional recordings are also possible and linearity is good although setup is slow and calibration is needed. A reasonable coil system can be bought for about $15,000; each eye-coil costs about $100. A typical eye coil lasts for two subjects. There is a small risk of a corneal abrasion from the contact lens. Only about 30 minutes of continuous recording is usually possible at one setting. Eye-coil systems are usually research tools. To use an eye-coil system subjects must sign consent because of the risk of corneal abrasion. This technology is certainly the most expensive of all, because of the cost of the eye-coils.
6. Video-Oculography (VOG)
Video-based eye movement recordings have become more and more popular because of the rapid progress made in electronic data processing. Most fundamental VOG techniques are based on tracking of the position of eye-fixed markers in a 2-D image. These positions have to be expressed in head-fixed coordinates. Since head-fixed markers are difficult to obtain with high precision, one strategy of VOG systems is to attach the video camera as
firmly as possible to the head. A translation of 1 mm will result in an error of about 5. Head-fixed devices cause a problem under head free conditions, because the stability of the head mount is not sufficient. Because of this problem, actual VOG systems can make highly accurate measurements of eye position, only as long as the head
is stable in space. A VOG method of compensating for head translation uses the relative position of the corneal reflex of an infrared LED (Eyelink IIR, SR Research, Osgode, Canada). One difficulty with this method is that using the corneal reflection adds more noise. For eye movements of about 12–15 the reflection reaches the edge of the cornea, and can no longer be used for compensation. Moreover, this approach relies on the topography of the cornea, which varies between subjects. Therefore, it seems to be useful when compensating for large translations, but may be unable to provide very high accuracy. Since the pupil position is detected and evaluated in image coordinates, the nonlinearity of the VOG systems (in contrast to IRD’s) is well defined by the geometry of the image projection. The main aim of the VOG calibration is therefore to determine the location of the center of rotation of the eye and the radius of the eyeball. The resolution of the 2-D VOG defined by the standard deviation of system noise measured with an artificial eye is about 0.01. The VOG of 2-D measurements of ocular torsion also reach accuracy values that are similar to those of coil measurements. The spatial resolution is 1 part in 1024 and now with high-speed cameras temporal resolution is as high as 1000 Hz. VOG can record vertical and torsion movements. Setup is very fast and calibration is necessary. Goggles effectively blackout vision so maintaining a “light-tight” lab is not crucial. This is very important. Systems costs from $18, 000 – $50,000.
Selected references
1. Eggert T. Eye movement recordings: methods. Dev Ophthalmol 2007;40:15-34.
2. Wade NJ, Tatler BW, Heller D. Dodge-ing the issue: Dodge, Javal, Hering, and the measurement of saccades in eye-movement research. Perception 2003;32:793-804.
3. Wade NJ. William Charles Wells (1757-1817) and vestibular research before Purkinje and Flourens. J Vestib Res 2000;10:127-37.
4. Smith CR. Measurement of nystagmus using electronystagmography (ENG). J Speech Hear Disord 1967;32:133-8.
5. Torok N. Measuring Eye Movements. Science 1960;131:940.
6. Robinson DA. A Method of Measuring Eye Movement Using a Scleral Search Coil in a Magnetic Field. IEEE Trans Biomed Eng 1963;10:137-45.
7. Henn V, Straumann D. Three-dimensional eye movement recording for clinical application. J Vestib Res 1999;9:157-62.
8. Traisk F, Bolzani R, Ygge J. A comparison between the magnetic scleral search coil and infrared reflection methods for saccadic eye movement analysis. Graefes Arch Clin Exp Ophthalmol 2005;243:791-7.
9. Houben MM, Goumans J, van der Steen J. Recording three-dimensional eye movements: scleral search coils versus video oculography. Invest Ophthalmol Vis Sci 2006;47:179-87.
10. Allum JH, Honegger F, Troescher M. Principles underlying real-time nystagmus analysis of horizontal and vertical eye movements recorded with electro-, infra-red-, or video-oculographic techniques. J Vestib Res 1998;8:449-63.
11. van der Geest JN, Frens MA. Recording eye movements with video-oculography and scleral search coils: a direct comparison of two methods. J Neurosci Methods 2002;114:185-95.
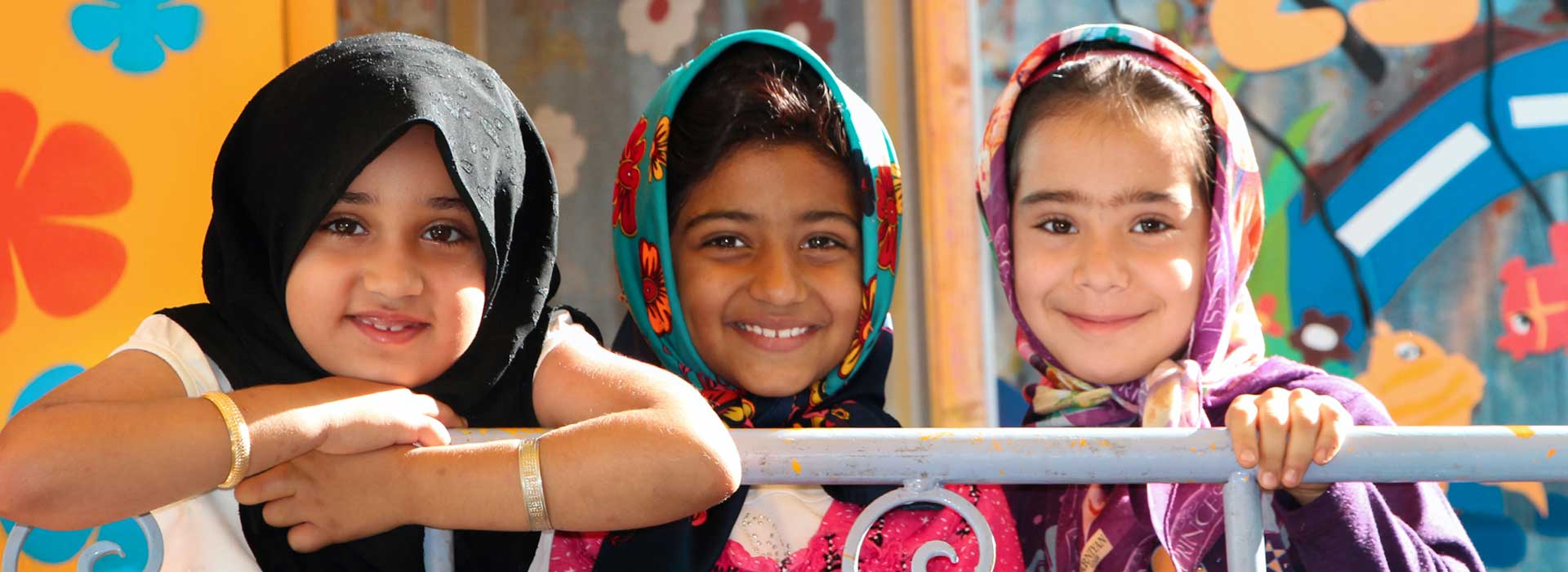